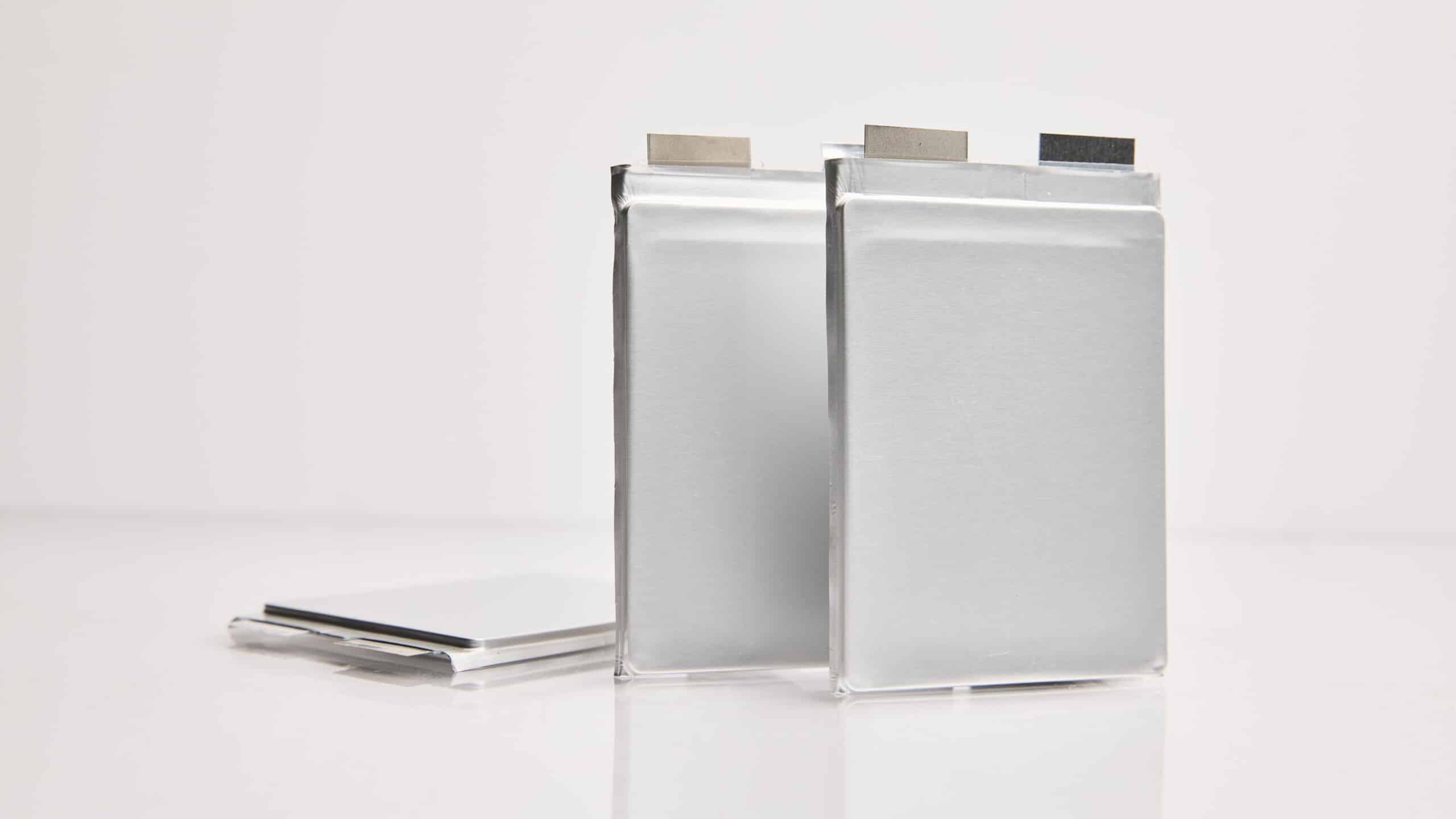
Our Strategic Blueprint
I took on the CEO role last year because I see QuantumScape as the global leader in solid state battery technologies with an ability to revolutionize energy storage and create tremendous shareholder value in the process.
Energy is one of the most important features of battery performance; it determines how long your smartphone can run or how far your electric vehicle can drive. One of the most valuable ways to assess the quality of a battery is by how much energy can fit in its size or weight, or its energy density. Higher energy density batteries can store more energy in a smaller or lighter package, making them more practical for certain applications where space or weight matter.
Yet, as with every other aspect of batteries, it’s not as straightforward as it may seem. There are many factors to consider when evaluating a battery’s energy density, some of which are not obvious. When energy density figures are quoted without context, it can be hard to tell whether a new battery cell is impressive or ordinary.
In this series of blog articles, we will attempt to illuminate the complexities behind this deceptively simple concept and hopefully make it easier to understand the possibilities and tradeoffs involved in building better, more energy-dense batteries. We will begin with a basic overview of energy density and continue with the other factors that affect a battery’s energy density:
One of the most critical things to understand about battery energy density is that there are two different ways to measure it – by volume and by weight. While they are sometimes conflated, they are significantly distinct and tell us different things about the battery’s performance capability.
The amount of energy that can fit in a given physical volume is most important for applications that are volume-constrained – where space matters. Consumer electronics and passenger vehicles are two good examples of this.
In addition, other popular electric vehicle types, like SUVs and pickup trucks, are already quite large, but due to lower aerodynamic efficiency, offer range that may not satisfy the needs of every driver. Since the vehicles themselves can’t easily be made larger to accommodate more batteries, the battery cells themselves need to pack more energy into the available space. Although weight is also important, for these use cases, better volumetric energy density is a bigger priority.
Weight is at an absolute premium for applications like drones or aerospace, . In these cases, a bulkier battery can be accommodated by good design, but increased weight always constrains performance, and so the lighter the battery, the better. In extreme cases, such as batteries in satellites, every extra kilogram of weight adds thousands of dollars to the cost of launch.
There are also other applications with more prosaic constraints. In heavy-duty trucking, for example, there are legal maximum weight limits to avoid damaging roadways. For a semi-truck, the math is simple: the less weight taken up by batteries, the more payload can be hauled, and the more the truck can earn per trip. In other use cases, like high-performance sports cars, lighter cars handle better and accelerate faster. For applications like these, gravimetric energy density, also known as specific energy, may represent the bigger pain point.
Battery energy density has slowly but steadily increased over the past few decades, and lithium-ion batteries are now so energy dense that they can power electric vehicles for hundreds of miles. After all that progress, what’s the need for an even better battery?
The truth is that, when it comes to energy storage, there is always a use case for better energy density. With better energy density:
Companies are always competing to offer a car or a laptop or a drone that outperforms the competition, and energy density represents one of the biggest bottlenecks to better performance. In short, better energy density is always in demand.
Beyond these direct performance benefits, improved energy density has potential side benefits as well:
How can battery energy density be improved? There are a few primary ways: using better active materials, optimizing the loading and porosity of the active materials, and implementing more efficient cell packaging. Continue reading to learn more.
Forward-Looking Statements
This article contains forward-looking statements within the meaning of the federal securities laws and information based on management’s current expectations as of the date of this current report. All statements other than statements of historical fact contained in this article, including statements regarding the future development of QuantumScape’s battery technology, the anticipated benefits of QuantumScape’s technologies and the performance of its batteries, and plans and objectives for future operations, are forward-looking statements. When used in this current report, the words “may,” “will,” “estimate,” “pro forma,” “expect,” “plan,” “believe,” “potential,” “predict,” “target,” “should,” “would,” “could,” “continue,” “believe,” “project,” “intend,” “anticipates” the negative of such terms and other similar expressions are intended to identify forward-looking statements, although not all forward-looking statements contain such identifying words.
These forward-looking statements are based on management’s current expectations, assumptions, hopes, beliefs, intentions, and strategies regarding future events and are based on currently available information as to the outcome and timing of future events. These forward-looking statements involve significant risks and uncertainties that could cause the actual results to differ materially from the expected results. Many of these factors are outside QuantumScape’s control and are difficult to predict. QuantumScape cautions readers not to place undue reliance upon any forward-looking statements, which speak only as of the date made. Except as otherwise required by applicable law, QuantumScape disclaims any duty to update any forward-looking statements. Should underlying assumptions prove incorrect, actual results and projections could differ materially from those expressed in any forward-looking statements. Additional information concerning these and other factors that could materially affect QuantumScape’s actual results can be found in QuantumScape’s periodic filings with the SEC. QuantumScape’s SEC filings are available publicly on the SEC’s website at www.sec.gov.
I took on the CEO role last year because I see QuantumScape as the global leader in solid state battery technologies with an ability to revolutionize energy storage and create tremendous shareholder value in the process.
QuantumScape’s planned first commercial product, QSE-5, is a ~5 amp-hour cell designed to meet the requirements of automotive applications. Here, we’ll walk through the various elements of the cell specifications and explain some of the complexities behind the seemingly simple metric of energy density.
I took on the CEO role last year because I see QuantumScape as the global leader in solid state battery technologies with an ability to revolutionize energy storage and create tremendous shareholder value in the process.
QuantumScape’s planned first commercial product, QSE-5, is a ~5 amp-hour cell designed to meet the requirements of automotive applications. Here, we’ll walk through the various elements of the cell specifications and explain some of the complexities behind the seemingly simple metric of energy density.
Privacy Policy | Terms of Use
© 2025 QuantumScape Battery, Inc.
1730 Technology Drive, San Jose, CA 95110
info@quantumscape.com
Pamela Fong is QuantumScape’s Chief of Human Resources Operations, leading people strategy and operations, including talent acquisition, organizational development and employee engagement. Prior to joining the company, Ms. Fong served as the Vice President of Global Human Resources at PDF Solutions (NASDAQ: PDFS), a semiconductor SAAS company. Before that, she served in several HR leadership roles at Foxconn Interconnect Technology, Inc., a multinational electronics manufacturer, and NUMMI, an automotive manufacturing joint venture between Toyota and General Motors. Ms. Fong holds a B.S. in Business Administration from U.C. Berkeley and a M.S. in Management from Stanford Graduate School of Business.